With a market cap of over half a trillion dollars, Tesla Motors has become the most valuable car company in the world. They achieved this by revolutionizing the automotive industry and pushing electric vehicles into the global consciousness. This impressive feat was accomplished despite facing off against automotive giants like Ford and Toyota. However, Tesla’s success would not have been possible without a critical element that has become the backbone of global electronics: lithium.
Dubbed “white gold”, lithium powers everything from cell phones and laptops to power tools, e-bikes, and even electric toothbrushes, making it a cornerstone of modern technology. Since their commercialization in the early 1990s, lithium-ion batteries have revolutionized energy storage, driving advancements that seemed like science fiction just a few decades ago.
More recently, it has become the backbone of the modern automobile. However, the surging demand for electric vehicles and the global shift towards renewable energy sources are putting immense pressure on lithium supplies. The International Energy Agency (IEA) predicts that by 2035, anticipated supplies of lithium will only meet 50 percent of worldwide demand.
There simply isn’t going to be enough lithium on the face of the planet, regardless of who expands and who delivers, it just won’t be there.
- Stuart Crow, chair of Lake Resources
Without the ability to meet demand, our dependence on lithium could spell trouble for both the technology and automotive industries, potentially stalling the growth of electric vehicles and renewable energy projects, and hampering efforts to reduce global carbon emissions. Without sufficient lithium, costs could rise significantly, putting the very foundation of the green-tech revolution at risk.
This soaring global demand for lithium is driving a mining boom in the Andes region of South America, where half of the world’s reserves are located. Lithium is extracted from two main sources: minerals and brines. In the arid salt flats of Argentina, Chile, and Bolivia, it is primarily extracted from brines.
The process is straightforward but time-consuming. Naturally salinated water is pumped to the surface from underground reservoirs and into a series of evaporation pools. Over many months or even years, the water gradually evaporates, leaving behind a thick brine rich in lithium carbonate.
Yet, this seemingly simple extraction process is a silent thief, consuming vast amounts of water vital for the delicate balance of local ecosystems. Although brine contains mostly water, its high salt concentration makes it unsuitable for drinking. Normally, we wouldn’t see this as an issue, but extracting lithium-rich brine disrupts the natural water cycle, depleting precious freshwater supplies that are essential for local communities and wildlife.
When rain falls on the nearby Andes mountains, it streams down into underground aquifers, taking minerals along with it. The older, heavier, and saltier brine sinks to the bottom, while the freshwater floats on top. When the aquifers are full, the freshwater on top creates lagoons above ground that are critical for the survival of local indigenous communities and many endangered species, including flamingoes. Extracting lithium-rich brine from these aquifers reduces the volume of water they contain, which in turn reduces the amount of accessible freshwater that can make it to the surface to form the lagoons.
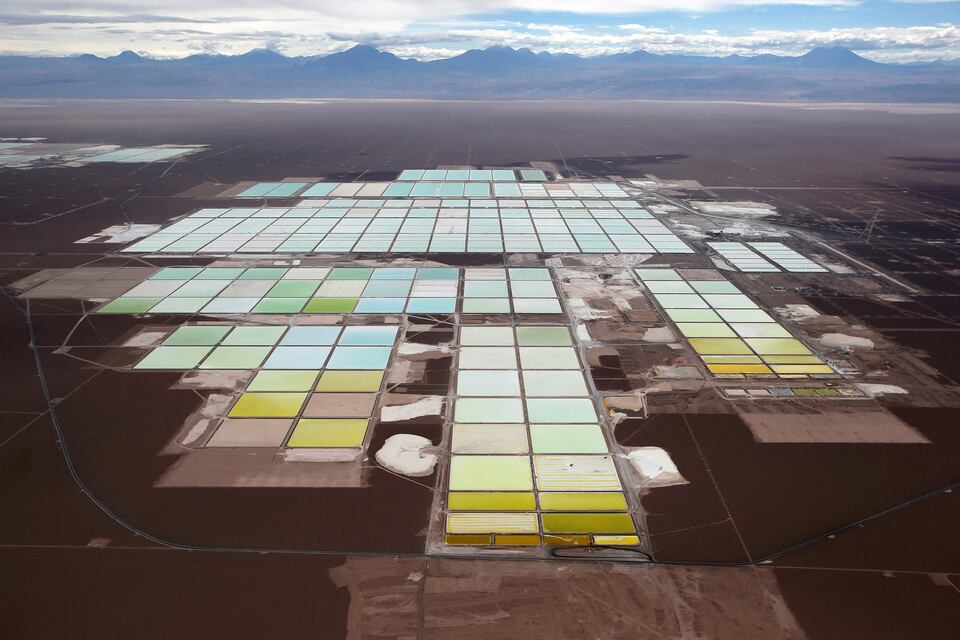
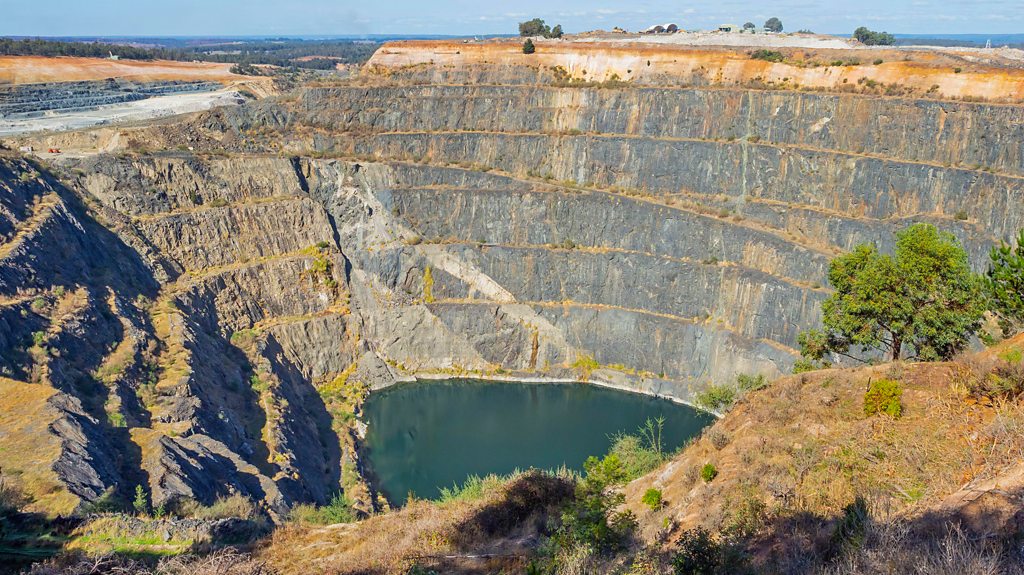
It takes half a million gallons of water to produce just one metric tonne of lithium from the salt flats, which is enough to make just fifteen batteries for the Tesla Model S. That means a single Model S needs 126,000 liters of water—enough water to sustain an adult man for 86 years—to create the 63 kilograms of lithium needed for its battery.
Chile’s Atacama salt flats produce more than a quarter of the world’s lithium but receive less than 15 millimeters of rain per year. It’s estimated that lithium mining activities consume 65% of the region’s water supply, which local communities and wildlife depend on.
Furthermore, toxic chemicals like hydrochloric acid are used in the extraction process and can leak from the evaporation pools into local freshwater supplies. The environmental impact of this method of lithium extraction is clearly significant.
Pound for pound, hard rock lithium mining is 16 times more carbon intensive than the dirtiest crude oil production.
Across the Pacific Ocean, Australia has the second largest lithium reserves in the world after Chile but is responsible for nearly half of global lithium production. While South American countries get nearly all their lithium from underground brine reserves in their salt flats, Australia’s reserves are locked away in mineral deposits in hard pegmatite rock that require more traditional mining methods for extraction.
The raw minerals mined from pegmatite typically contain around 1-2 percent lithium. The rock is then crushed and chemically roasted to produce a concentrated lithium ore called “spodumene,” which contains about 6 percent lithium. The material is then shipped to China, where 60 percent of the world’s lithium is currently refined—though that may soon change as Western nations, including Australia, seek to diversify their supply chains.
Hard rock mining invariably uses vast amounts of fossil fuels to power the machines used for digging and crushing rock. Processing spodumene also requires a significant amount of energy, and anywhere from 300,000 to 500,000 liters of water per tonne of lithium produced. Additionally, much of this processing occurs in China, where the electric grid relies heavily on coal and other fossil fuels, resulting in significant carbon dioxide emissions.
Overall, about 15 tonnes of carbon dioxide is emitted for every tonne of lithium produced. Pound for pound, hard rock lithium extraction emits more than 16 times more carbon dioxide than extracting oil from the Athabasca oil sands in Alberta, Canada, which is considered among the most carbon-intensive operations in the world.
The reason we don’t hear much about the carbon intensity of lithium mining is due to the relatively smaller volume of its impact. In 2022, Australia produced 61,000 tonnes of lithium for the entire year. In stark contrast, Alberta’s oil sands were producing 3.6 million barrels of oil—amounting to 490,000 tonnes—every single day. This adds up to nearly 179 billion tonnes of oil annually, making Australia’s yearly lithium mining emissions a mere 0.5 percent of the emissions produced by the oil sands.
Figure 1 - Comparing CO2 emissions from oil vs. lithium production
- "Hard rock mining releases 15 tonnes of CO2 for every tonne of lithium."
- "Domestic crude oil weighs about 7.21 pounds per gallon, a barrel of oil weighs around 300 pounds or about 136 kilograms."
- "The least carbon-intensive [oil] operations produced the equivalent of 39 kilograms of carbon dioxide per barrel, while the most intensive produced 127 kilograms."
- 1 tonne (1000 kg) ÷ 136 kg per barrel = 7.35 barrels (equivalent to a tonne of oil)
- 7.35 barrels per tonne × 127 kg of CO2 emissions per barrel = 934 kg of CO2 emissions per tonne of oil
- 15,000 kg (CO2 emitted per tonne of lithium mined) ÷ 934 kg = 16.06 times more emissions than oil production
Pound for pound, Lithium mining is 16 times more carbon intensive than the dirtiest crude oil production.
A Cleaner Source of Lithium
Both of the methods currently used to extract lithium have a high environmental cost, but demand is only going to rise in the coming years as EVs become more popular. Consequently, the environmental impact of lithium extraction is poised to grow alongside the increasing demand for EVs. While hydrogen technology offers some solutions, it cannot replace the need for efficient, rechargeable batteries that are integral to modern civilization. Scientists and engineers worldwide are developing increasingly efficient batteries, but our dependence on lithium isn’t going away. So what can we do?
Scientists estimate that our oceans contain around 200 billion tonnes of lithium, which could provide a near-limitless supply of this critical metal to meet global demand. The problem is that the lithium concentration in seawater is very low—about 0.18 parts per million (ppm)—making traditional extraction methods, like evaporation pools, impractical. Brine needs to have at least 50 ppm to be viable for extraction.
Researchers at King Abdullah University of Science and Technology in Saudi Arabia may have discovered a cost-effective way to concentrate the lithium in ocean water, potentially making it one of the most environmentally friendly methods of lithium extraction. Their innovative solution employs an electrochemical cell with a porous membrane that allows lithium ions to pass through while blocking unwanted elements.
Without delving into the technical details, an electrochemical reaction drives the lithium ions in seawater through the membrane, where they accumulate in a chamber. After several cycles, the lithium-enriched water can reach concentrations of more than 9,000 ppm. According to the researchers, it would take just $5 of electricity to produce one kilogram of lithium using this approach, making it cost-competitive with current brine extraction methods.
Moreover, this process also produces hydrogen and chlorine gas as byproducts, which can be captured and sold to offset electricity costs, further reducing production expenses. Extracting lithium from seawater would have minimal impact on local ecosystems, and if the electricity used comes from renewable sources like solar and wind, there would be no carbon emissions. While this process has yet to be proven at scale, the potential benefits are clear.
By one estimate, the cost of recycled lithium is five times that of virgin lithium from brine-mining. With growing EV sales, a massive wave of dead electric car batteries will soon exacerbate recycling problems.
- Ula Chrobak, Knowable Magazine
Yet even if we secure a limitless supply of lithium to power EVs far into the future, one significant problem remains. Globally, an estimated average of only 5 percent of lithium-ion batteries are recycled, and in some regions, the rate is even lower. It is currently cheaper to mine new lithium than to recycle existing batteries, resulting in the vast majority ending up in landfills where they decay and leak chemicals that contaminate groundwater and surface water.
Non-rechargeable alkaline batteries can be simply shredded, melted down, and recycled through a straightforward process. In contrast, lithium-ion batteries are more complex to recycle and can potentially explode if improperly handled. In 2021, there were 16.5 million EVs on the road, and if lithium supplies meet demand, that number is expected to balloon to 350 million by 2030. In 15 to 20 years, when all of those EV batteries reach the end of their lifecycle, we will face a significant problem if the infrastructure for recycling them is not in place.
While securing a cleaner and more sustainable source of lithium is crucial, it is not the only solution to the growing demand for batteries. Given the environmental and supply chain challenges associated with lithium, researchers around the world are exploring alternative battery technologies that could reduce our dependence on this critical resource. These alternatives hold the promise of not only easing the pressure on lithium supplies but also offering unique advantages in terms of cost, safety, and performance.
The Future of Battery Innovation
To address these challenges, scientists are developing and testing a variety of new battery technologies that don’t rely solely on lithium. Among the most promising are solid-state, sodium-ion, aluminum-ion, and magnesium-ion batteries. Each of these technologies offers distinct benefits that could revolutionize energy storage and reduce our reliance on lithium. However, despite their potential, these technologies have yet to become mainstream. Let’s explore how these innovative batteries work, their advantages, and the hurdles they face before they can power our future.
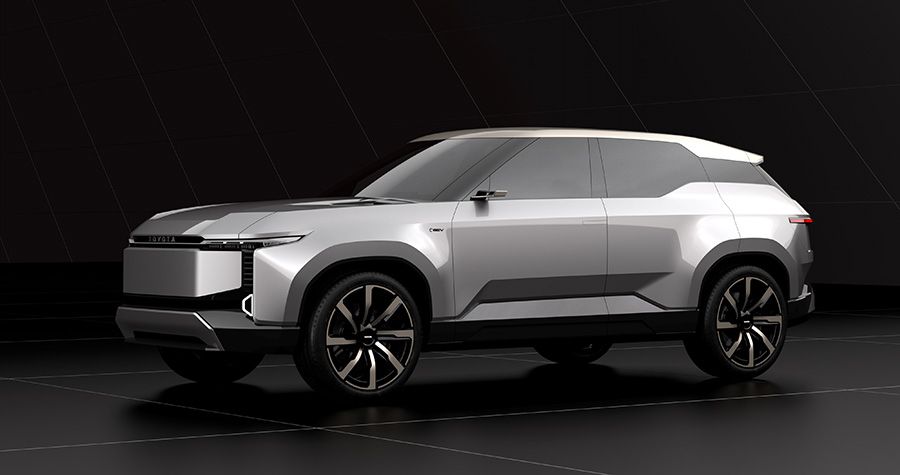
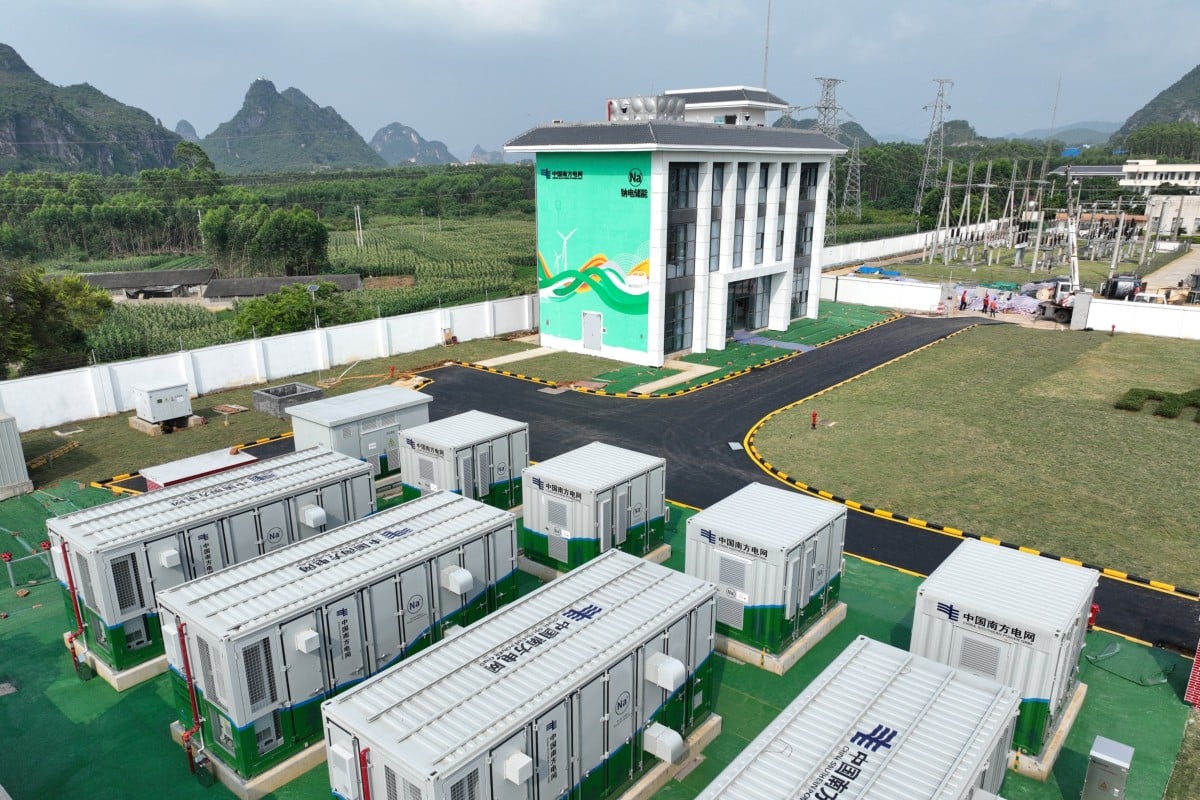
Solid-State Batteries
All batteries use chemistry to store electrical energy in the form of chemical potential. Every battery follows the same general design: two electric terminals separated by an electrolyte. The positive terminal is called the cathode, while the negative terminal is the anode. The electrolyte, a chemical material, allows an electric charge to pass between the two terminals.
When the battery is connected to an electric circuit, electrons flow from the anode to the cathode through to the external circuit, providing electrical energy to the connected device. In a rechargeable battery, electrons can move in either direction through the circuit and electrolyte, allowing the battery to both accept and release energy.
One of the biggest drawbacks of today’s lithium-ion batteries is their liquid electrolyte, which requires them to operate within a relatively narrow temperature range. In cold weather, the electrolyte’s viscosity increases, making it harder for ions to move between the terminals. This reduces the battery’s ability to accept and release energy, thereby lowering its overall capacity and making it harder to charge.
The liquid electrolyte is also flammable. If the battery is damaged or overheats (due to hot weather, overcharging, or other factors), an exothermic chemical reaction can occur within the electrolyte. This can cause a chain reaction, leading to the battery cell temperature rising up to around 400°C extremely quickly—a phenomenon known as “thermal runaway.” This can result in the battery melting, catching fire, or even exploding.
Solid-state batteries, which still use lithium as their core chemistry, represent a significant leap forward in battery technology by replacing the liquid electrolyte with a solid electrolyte. This change promises a range of benefits, from improved safety to higher energy densities and longer cycle life.
Toyota recently confirmed that solid-state battery technology offers significantly higher energy densities, allowing an electric vehicle to be charged in under 10 minutes with 1,200 kilometers (750 miles) of range on a single charge. They are also much safer because they eliminate the flammable liquid electrolyte, reducing the risk of fires and explosions. Toyota is aiming to launch a new line of EVs using solid-state batteries by 2028.
However, solid-state batteries are currently very expensive to produce due to the complex manufacturing processes required. The technology is still in the developmental stages, and scaling up production to meet commercial demands remains a significant challenge. Moreover, they still depend on lithium since a workable alternative has yet to be developed. So, while the technology is more efficient and safer, it doesn’t eliminate our need for lithium. We still need to explore further alternatives.
Sodium-ion Batteries
Sodium is the sixth most abundant element on Earth, with roughly 1,200 times more sodium available for extraction than lithium. Sodium-ion batteries are gaining attention as a potential alternative to lithium-ion batteries due to the abundance and low cost of sodium.
Unlike lithium, which is relatively scarce and geographically concentrated, sodium is widely available and inexpensive, making it an attractive option for large-scale energy storage. Sodium carbonate costs around $425 USD per tonne, a mere fraction of the $20,000 USD per tonne that lithium carbonate commanded in May 2024.
Sodium also requires far less water to produce, and batteries made with it are much easier to recycle, making it a more environmentally friendly option. Producing one tonne of lithium requires 682 times more water than producing one tonne of sodium.
Furthermore, sodium-ion batteries are much safer than lithium-ion batteries. They are less prone to overheating and thermal runaway, leading to fewer fires and explosions. The technology allows sodium-ion batteries to be completely discharged, ensuring they aren’t holding any current, which makes them safer for storage and transportation. They can also operate effectively at much lower temperatures, reportedly retaining 90 percent of their capacity in -20°C temperatures.
Scaling production of sodium-ion batteries would also be straightforward, as existing manufacturing facilities for lithium-ion batteries can be easily repurposed. Switching the type of battery produced would be relatively simple.
BloombergNEF predicts that sodium-ion batteries will make up 12% of the market for stationary energy storage by 2030.
- David R Baker, Bloomberg
However, there are some drawbacks to this technology. One of the main challenges with sodium-ion batteries is their lower energy density. They can’t store as much energy per unit weight compared to lithium-ion batteries, which limits their use in high-demand applications like electric vehicles. They also typically have a shorter cycle life, meaning they can undergo fewer charge and discharge cycles before their capacity degrades.
Despite these limitations, sodium-ion batteries could be ideal for stationary energy storage applications, such as balancing supply and demand on the electrical grid, where they could completely eliminate the need for lithium. However, as we continue to search for the perfect replacement for lithium, another promising contender is aluminum-ion batteries. Utilizing aluminum as the charge carrier, these batteries offer unique advantages that could further revolutionize the future of energy storage.
Aluminum-ion Batteries
Aluminum-ion batteries are another promising alternative, utilizing aluminum as the charge carrier. Aluminum is abundant, inexpensive, and has three valence electrons compared to lithium’s one. This means each aluminum ion is capable of carrying three electrons, while lithium ions can only transfer one. This gives aluminum the potential for much higher charge storage capacity, making it a suitable option for EV batteries.
Aluminum is the most abundant metal and the third most abundant chemical element on Earth. It is already widely used in virtually every industry, making sourcing the necessary material simple and inexpensive. Market forces can affect the price of aluminum, but it tends to go for around $2,500 to $3,000 USD per tonne, making it approximately 85 percent cheaper than lithium.
Aluminum is also easily recyclable and there is no limit to the number of times it can be recycled. There is extensive infrastructure already in place to recycle it, and the automotive and construction industries already recycle 90 percent of used aluminum. Recycled aluminum requires 95 percent less energy than primary aluminum production, significantly reducing its carbon footprint.
The graphene aluminum-ion battery cells from the Brisbane-based Graphene Manufacturing Group (GMG) are claimed to charge up to 60 times faster than the best lithium-ion cells.
- Michael Taylor, Forbes
While the aluminum-ion batteries developed thus far are only half as energy dense as lithium-ion ones, in principle, aluminum is a significantly better charge carrier than lithium. Recent breakthroughs have made it possible for aluminum-ion batteries to charge 60 times faster than lithium-ion batteries, allowing them to fully charge in just 6 minutes.
Battery lifespan has also greatly improved, with aluminum-ion batteries retaining 88 percent of their capacity after 5,000 cycles, even when using high voltage current to charge the battery quickly. In contrast, lithium-ion batteries can lose 20 percent or more of their capacity after just 500 cycles.
While aluminum-ion batteries offer many advantages, such as their ability to charge extremely quickly, potentially higher capacity, and improved safety, more research is needed to achieve higher energy density and voltage so they can compete with lithium-ion batteries.
Aluminum production was also responsible for about 3 percent of the world’s direct industrial CO2 emissions in 2022, which hinders its potential to become an environmentally friendly alternative to lithium. Despite these challenges, the potential of aluminum-ion batteries to transform the energy storage landscape is undeniable.
As researchers continue to explore and enhance aluminum-ion technology, another exciting candidate is emerging on the horizon: magnesium-ion batteries. These batteries promise to offer even greater benefits and could further revolutionize the future of energy storage.
Magnesium-ion Batteries
A promising contender that could reduce or even eliminate our dependence on lithium is the magnesium-ion battery. Magnesium is almost as abundant as sodium, making it significantly more plentiful than lithium.
Magnesium is a silvery-white metal, similar in appearance to aluminum, but it is only two-thirds the weight. While aluminum is primarily produced from bauxite ore extracted from open-pit mines, magnesium can be recovered from magnesium chloride, which is found abundantly in salt-water lakes and, more importantly, seawater. After sodium, it is the most abundant element found in our oceans, and it can be recovered with minimal environmental impact using electrolysis.
Magnesium is present in seawater at about 1,300 ppm, significantly higher than lithium’s 0.18 ppm, and it can also be found in rivers at 4 ppm. While the market price for magnesium can vary, it tends to trade for around $3,000 per tonne, making it more expensive than aluminum but still far cheaper than lithium.
Although seawater is only approximately 0.13 percent magnesium, it represents an almost inexhaustible source.
Researchers have found that, compared to their lithium-ion counterparts, magnesium-ion batteries offer several significant advantages. These batteries are cheaper to produce, boast a higher energy density—meaning smaller batteries with the same energy output—are more stable and less prone to explosion, utilize raw materials that are far more abundant around the world, and have the potential to last substantially longer.
However, there are still technological hurdles to overcome before magnesium-ion batteries can be commercialized. The primary challenges involve finding the right chemical and material composition to deliver enough voltage and capacity to compete with lithium-ion batteries. Overcoming these challenges could make magnesium-ion batteries an environmentally friendly solution for EV batteries that improves upon lithium-ion technology in almost every way.
Magnesium-ion batteries hold significant promise for the future of electric vehicles. If researchers can solve the existing technological challenges, these batteries could offer a more sustainable and cost-effective solution compared to lithium-ion batteries. Their higher energy density means that EVs could potentially travel longer distances on a single charge, and their improved safety profile could make them a more reliable option for consumers.
Despite their potential, magnesium-ion batteries are still in the research and development phase. It may take several more years of intensive research and testing before these batteries are ready for commercial use. Nevertheless, ongoing advancements in this field provide hope for a future where magnesium-ion batteries could play a crucial role in reducing our dependence on lithium and powering the next generation of electric vehicles.
Final Thoughts
The race to find sustainable solutions for our energy needs is more critical than ever, especially as the demand for electric vehicles and renewable energy sources continues to surge. While lithium-ion batteries have driven significant advancements in technology, the environmental consequences and supply chain challenges associated with lithium extraction and production cannot be ignored. Exploring alternative methods of extracting lithium and developing new battery technologies that don’t rely on lithium offers promising paths forward.
These innovative technologies not only have the potential to mitigate the environmental impact of lithium mining but also promise to deliver safer, more efficient, and more abundant energy storage solutions. While each technology comes with its own set of challenges, ongoing research and development efforts are paving the way for a future where our dependence on lithium could be significantly reduced.
By diversifying our energy storage solutions and embracing these cutting-edge innovations, we can create a more resilient and sustainable energy infrastructure. The journey towards a greener future is complex, but with continued dedication and innovation, we have the tools and knowledge to make it a reality.