Here are the key points:
- Battery EVs face limitations:
- Long charging times and range anxiety.
- Battery performance degradation in hot and cold climates.
- Fossil fuels still generate most grid electricity used to charge batteries.
- Mining lithium, cobalt, and nickel for battery production has a negative environmental impact.
- Battery capacity degrades over time and replacements are expensive.
- Hydrogen Fuel Cells have advantages that are needed in order to address BEV limitations and drive EV adoption in key markets:
- Quick refueling times (3-5 minutes).
- Consistent performance in extreme hot and cold climates.
- Hydrogen generates completely clean energy, with just water and heat as byproducts.
- Less environmental impact from production compared to batteries.
- Longer operational life and higher energy density than batteries.
In 1996, Elon Musk was still a student at Stanford University, and Tesla was just the last name of one of history’s greatest inventors. Meanwhile, General Motors began production on a vehicle that would forever alter the landscape of the automobile industry—the EV1. The EV1 was the first purpose-built electric vehicle of the modern era, utilizing rechargeable lead-acid batteries. Although this cutting-edge vehicle was intended for mainstream consumers, it was only available for lease; ownership was not an option.
Despite favorable customer reception, GM ultimately declared the EV1 unprofitable and ended its production by 1999. Instead of allowing customers to purchase the vehicles—which many were willing to do—GM collected all the leased units and had them crushed.
Many have questioned why GM chose to eliminate the EV1 rather than let it thrive in the market. The prevailing theory is that the oil and gas industry felt threatened by its potential and wanted the technology buried, while GM itself was concerned about losing revenue from spare parts, as electric vehicles require far less maintenance than their gas-powered counterparts.
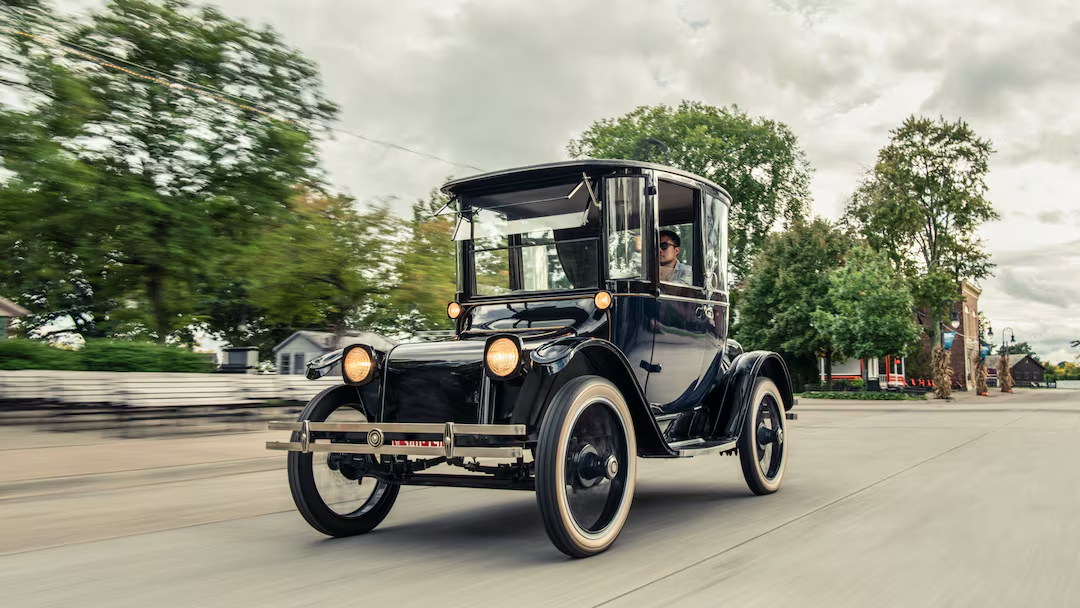
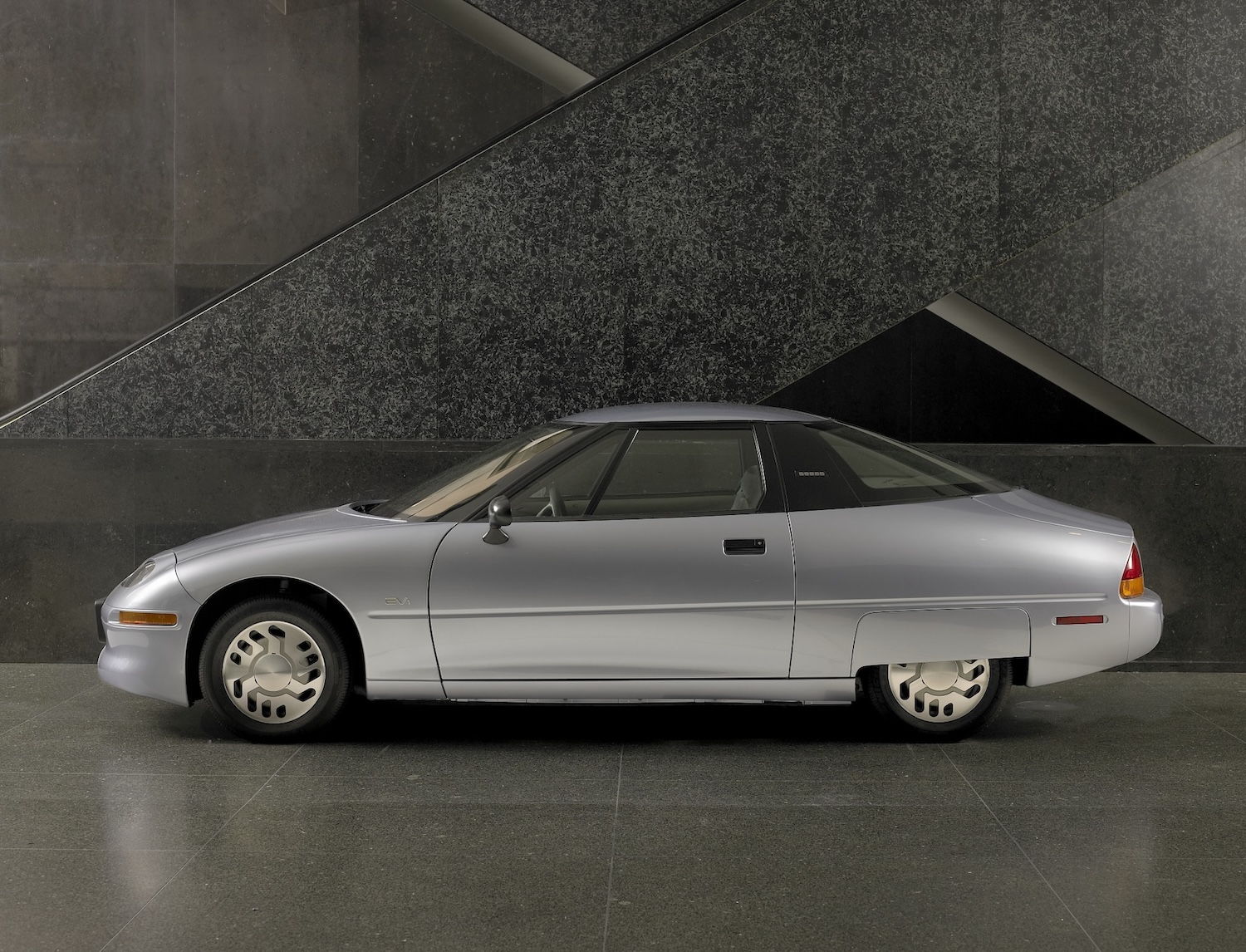
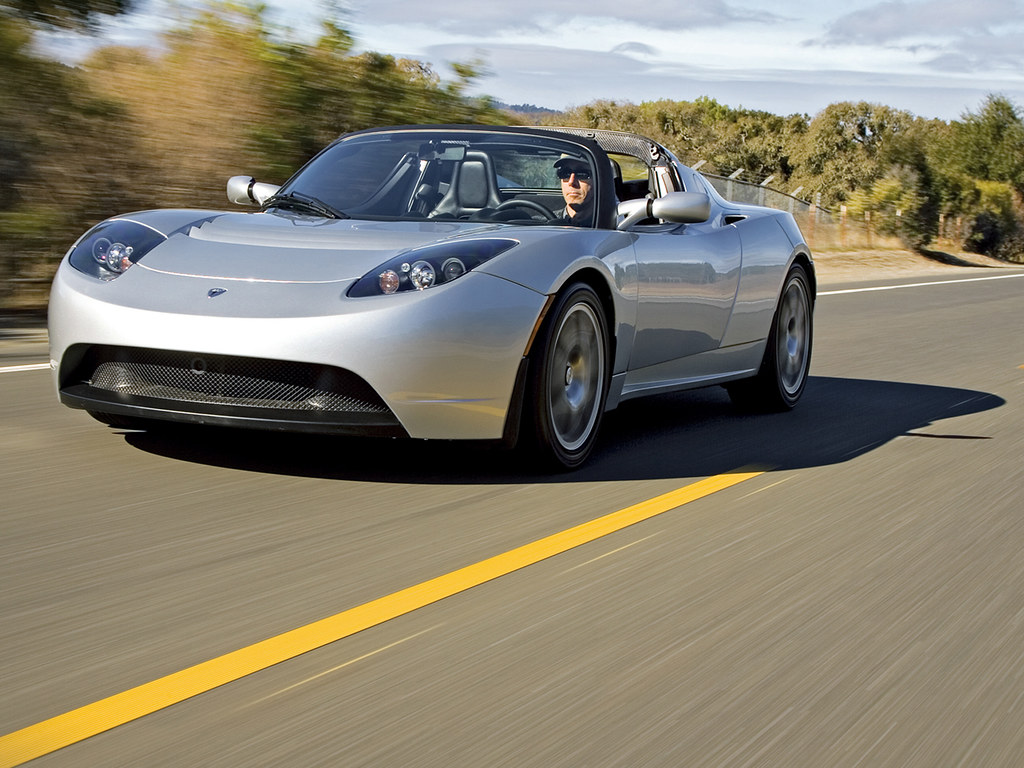
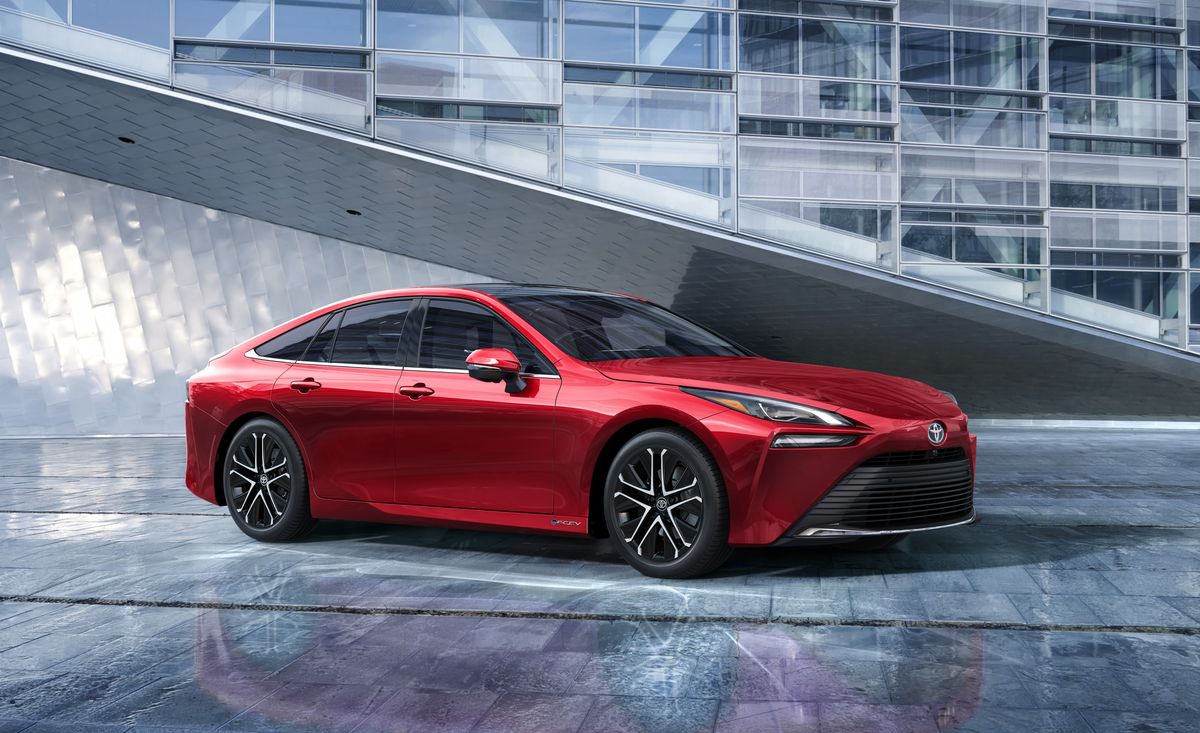
The demise of the EV1 ultimately inspired what would become a revolution in the automotive industry. Shortly after the EV1 had been discontinued, two engineers from California, Martin Eberhard and Marc Tarpenning, wanted to build a new kind of car company that was also a technology company. Named in tribute to the great Serbian-American inventor, Tesla Motors was incorporated in July 2003.
In February 2004, Elon Musk joined the company as its largest investor and shareholder. By October 2008, internal politics led to the departure of Eberhard and Tarpenning, and Musk assumed the role of CEO. That same year, Tesla began production on their flagship vehicle, the all-electric Roadster, capable of accelerating from 0–60 mph in just 1.9 seconds, making it one of the fastest cars in the world.
Since then, the automotive world has been abuzz with the promise of a revolutionary technology: the lithium-ion battery electric vehicle (BEV). Tesla captivated the public with sleek designs, impressive ranges, and the tantalizing prospect of a zero-emission future. Governments and consumers alike embraced BEVs, leading to a surge in sales and innovation that has made Tesla the most valuable car brand in the world, while simultaneously forcing a reluctant automotive industry into an electrified future.
The early adopters have all, well, adopted. And everyone else isn’t feeling that willing to take risks in their car buying.
- Jessica Caldwell, director of insights at Edmunds
Yet, as we approach the mid-2020s, the initial excitement has begun to wane, revealing cracks in the facade of the BEV utopia. In 2023, EV sales began to plateau and that trend is continuing in 2024. As the market transitions into a new phase, consumers are beginning to question the value of EVs.
While BEVs offer an emission-free driving experience and the ability to “fuel up” at home, they still grapple with practical issues like long charging times and limited range, leading to “range anxiety” among potential buyers. The infrastructure for charging remains unevenly distributed, particularly in rural areas, making BEVs less accessible for many.
Moreover, the environmental cost of lithium-ion battery production is staggering. Mining for lithium, cobalt, and nickel depletes natural resources and devastates ecosystems, often in developing countries where regulations are lax. Furthermore, the energy required to produce and recycle these batteries can offset the emission savings gained from driving an electric car. These shortcomings highlight a critical need for an alternative that can complement and eventually surpass BEVs in the quest for sustainable transportation.
But first, let’s dig into those limitations.
The Limitations and Drawbacks of Batteries
Battery electric vehicles have undoubtedly revolutionized the automotive industry. They offer a cleaner driving experience and the cost of electricity is much cheaper than fueling up at the gas station. The ability to charge a BEV at home is a game-changer and is a significant part of their appeal.
BEVs also feature impressive acceleration, quiet operation, and lower maintenance costs compared to traditional internal combustion engine vehicles. This technological advancement has pushed the boundaries of what’s possible in clean transportation and has inspired significant infrastructure investments worldwide.
However, despite these obvious benefits, the lithium-ion batteries that power the new generation of EVs have some significant limitations. Range anxiety and battery life are two of the most common criticisms of BEVs.
Batteries lose capacity over time
Anyone who has owned a smartphone has experienced the gradual degradation of the battery’s capacity over time. Lithium-ion batteries in EVs are just larger versions of the kind used in electronics, like smartphones and laptops. These batteries can only be recharged a few hundred times before they start losing capacity. After around a thousand charge cycles, high-end lithium-polymer batteries can lose about 20 percent of their capacity.
Thanks to innovations in battery technology, BEVs are able to travel hundreds of kilometers on a single charge. However, it still takes hours to charge an EV battery using conventional charging stations. While fast-charging stations like Tesla’s superchargers can use high voltage current to charge an EV in about 15-30 minutes, they accelerate the battery’s degradation with each use, shortening its lifespan.
Since EV batteries lose their capacity as the vehicle ages, used BEVs may be harder to sell since it’s difficult to understand the condition of a used battery. The battery is also the single largest expense for an EV, and replacing one can cost upwards of $10,000 USD. While an internal combustion engine can be maintained and repaired, a battery cannot.
The high cost of replacing EV batteries has been making headlines, like a 2014 Ford Focus Electric bought for US$11,000 needing a US$14,000 battery.
- “Ontario man quoted $50,000 to replace an EV battery” by Lorraine Sommerfeld, Driving.ca
Temperature can greatly affect battery performance
Batteries, like humans, are quite sensitive to extreme temperatures. Lithium-ion batteries perform at their best in temperatures between 15 and 35 degrees Celsius, but most regions experience temperatures outside this range depending on the season.
When temperatures drop below freezing, resistance increases in the battery’s internal electrolyte fluid, making it harder to release energy or hold a charge, thus reducing the battery’s capacity. To prevent these problems, the battery needs to be kept warm in cold conditions.
However, unlike internal combustion engines that generate their own heat, EVs must source this heat elsewhere, typically by running a heater, which consumes electricity that could otherwise be used to power the vehicle. Consequently, battery-powered vehicles often lose 12% of their range in cold weather, and this number can soar to over 40% when the heater is on full blast.
At 5F (-15C), EVs drop to 54% of their rated range, meaning a car that is rated for 250 miles (402 km) will only get on average 135 miles (217 km).
- Charlotte Argue, Senior Manager of Sustainable Mobility at Geotab
Extreme heat presents similar challenges. When temperatures rise above 35°C, the battery loses charge faster, resulting in reduced range. Batteries function at peak condition at around 21-22°C, which also happens to be the temperature range where humans feel most comfortable.
When a car’s interior temperature drops below 20°C, we tend to turn on the heat. Likewise, when the temperature rises above 23°C we tend to turn on the air conditioning. When we turn on the heat or the air conditioning in a BEV, however, we’re consuming energy that is also used to move the car, further reducing its range.
EVs use thermal management systems designed to maintain the battery’s ideal temperature range for optimal performance, which has the added benefit of preventing degradation. However, while these systems draw energy to warm or cool the battery, they also heat or cool the car’s interior for the occupants, which accelerates battery drain as weather conditions become hotter or colder.
These factors affect the range of gas-powered vehicles as well, but if you run low on fuel, you can just stop at any one of a plethora of gas stations and have a full tank again in less than five minutes. You aren’t stuck charging your battery for 20-30 minutes, provided you can even find a fast-charging station in the first place.
Battery fires are difficult to extinguish
Safety is another significant concern with lithium-ion vehicles. If the temperature reaches extremes, the battery runs the risk of igniting and causing an explosion. This can happen spontaneously, but becomes considerably more likely after a vehicle collision. As of May 26th, 2024, there had been 232 confirmed cases of Tesla vehicles catching fire, and 83 people have died when a battery fire was involved.
Chemical fires from lithium-ion batteries are very difficult to extinguish and are prone to reigniting after they’ve stopped burning. The lithium salts in the battery are self-oxidizing, which means they can’t be smothered and starved of oxygen like a conventional fire. Battery fires pose an added burden for firefighters, as they require considerably more time and resources to extinguish.
In fact, since the early 1990s, hundreds of passenger and cargo planes have suffered a fire or explosion due to the lithium-ion batteries that they were carrying.
- Melissa Hampton, Dangerous Goods Storage Consultant for Storemasta
The Environmental Impact of Lithium
One of the more obvious environmental problems with batteries is that most of the world still generates its electricity using fossil fuels. The U.S. and China, for example, are the largest greenhouse gas emitters in the world, and they both still generate nearly two-thirds of their electricity from fossil fuels. When an EV charges its battery using electricity generated from coal, it’s even worse for the environment than gasoline.
Regardless, lithium-ion batteries are still a crucial component of efforts to reduce our dependence on hydrocarbons. The battery of a Tesla Model S contains about 12 kilograms of lithium, but utility-scale batteries used with renewable energy systems require much more.
But there’s a problem. As the world scrambles to replace fossil fuels with clean energy, the environmental impact of sourcing all the lithium required for this transformation could become a serious issue in its own right.
One of the biggest environmental problems caused by our endless hunger for the latest and smartest devices is a growing mineral crisis, particularly those needed to make our batteries.
- Christina Valimaki, Analyst at Elsevier | WIRED Interview
In South America, the Lithium Triangle, which covers parts of Argentina, Bolivia, and Chile, holds more than half the world’s supply of the silvery-white metal beneath the salt flats. It’s also one of the driest places on Earth. This poses a real issue because extracting lithium involves drilling a hole in the salt flats and pumping salty, mineral-rich brine to the surface.
It’s a relatively cheap and effective process, but it uses a lot of water—approximately 500,000 gallons per tonne of lithium. In Chile’s Salar de Atacama, mining activities consumed 65 percent of the region’s water supply. This has a huge impact on local farmers, who grow quinoa and herd llamas, in an area where some communities already have to get water driven in from elsewhere.
To process lithium salts into a form that can be sold, they are mixed into huge evaporation pools filled with toxic chemicals, including highly corrosive hydrochloric acid, and left to dry out over many months. During the evaporation process, these toxic chemicals can potentially leak into nearby water supplies, which actually happened in Tibet in 2016.
The current methods for mining lithium are terrible for the environment, and we need to find alternative methods for obtaining the lithium we need relatively soon. In 2020, global demand for lithium was 292 thousand metric tonnes. By 2030, demand for lithium is expected to grow to 2.5 million metric tonnes, spurred by the increasing demand for electric vehicles.
The world is expected to need more than 11 million tonnes of lithium per year by 2050. With just 89 million tonnes of estimated global reserves, we would completely run out of lithium by 2047 if we don’t find more.
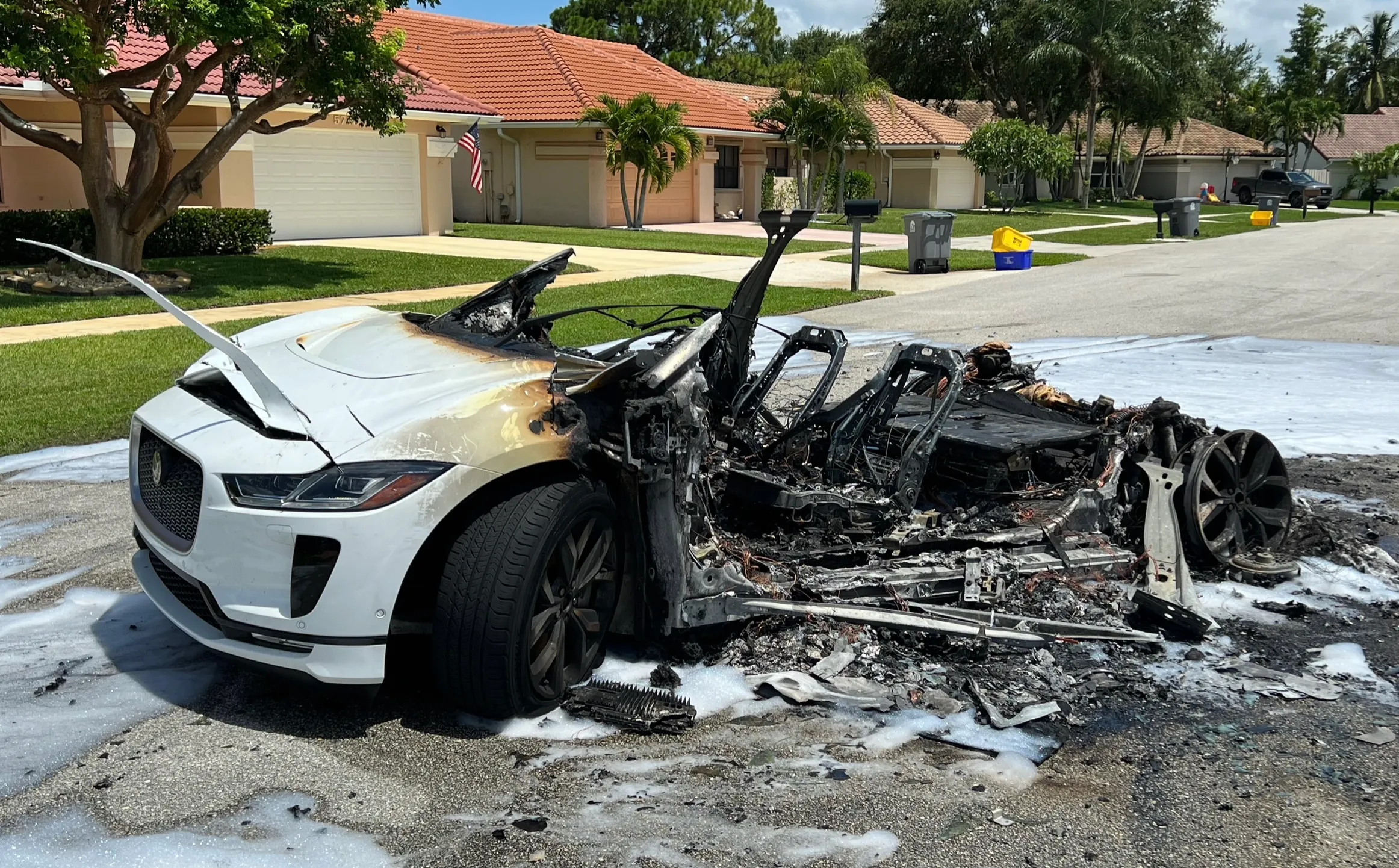
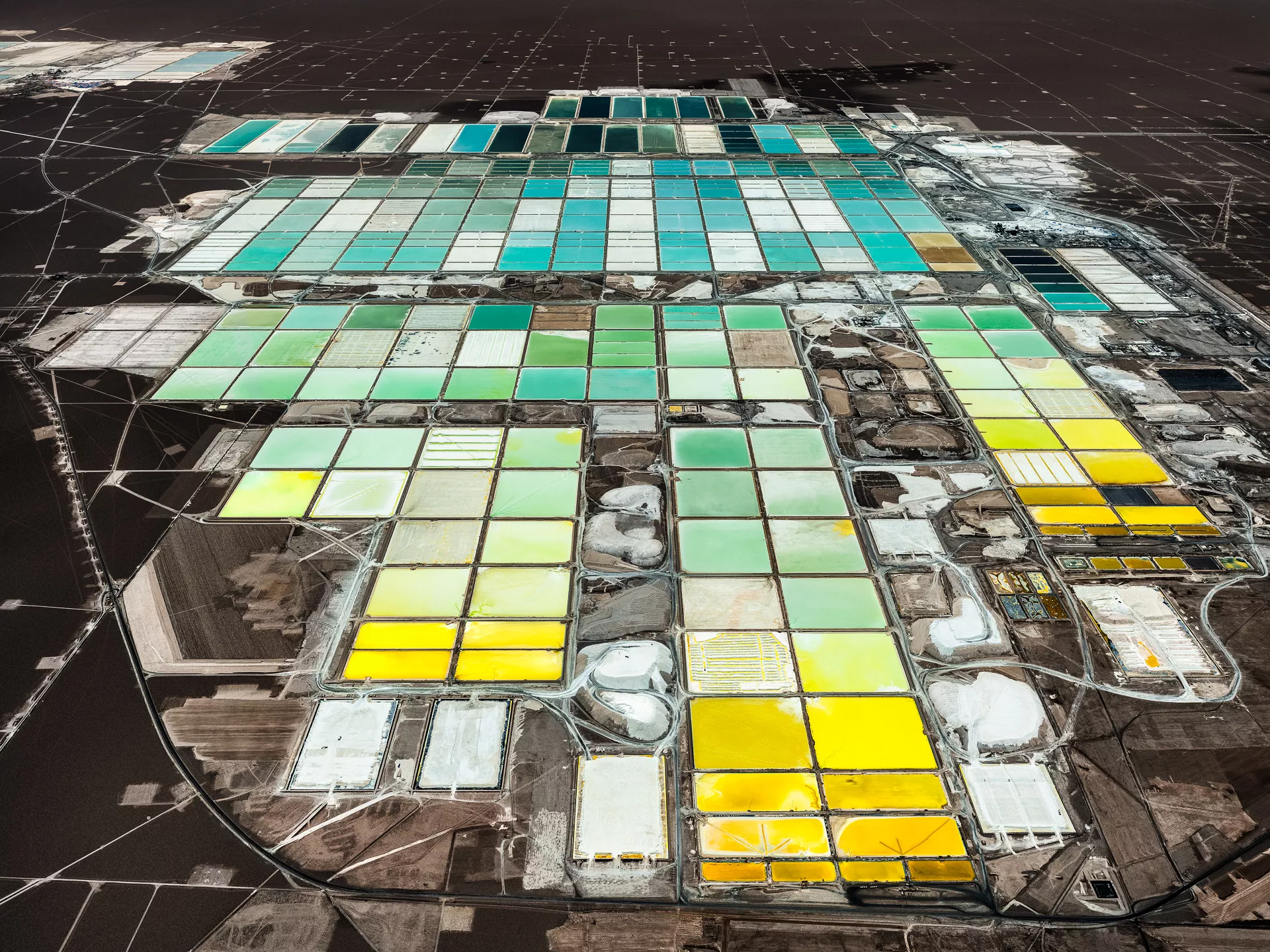
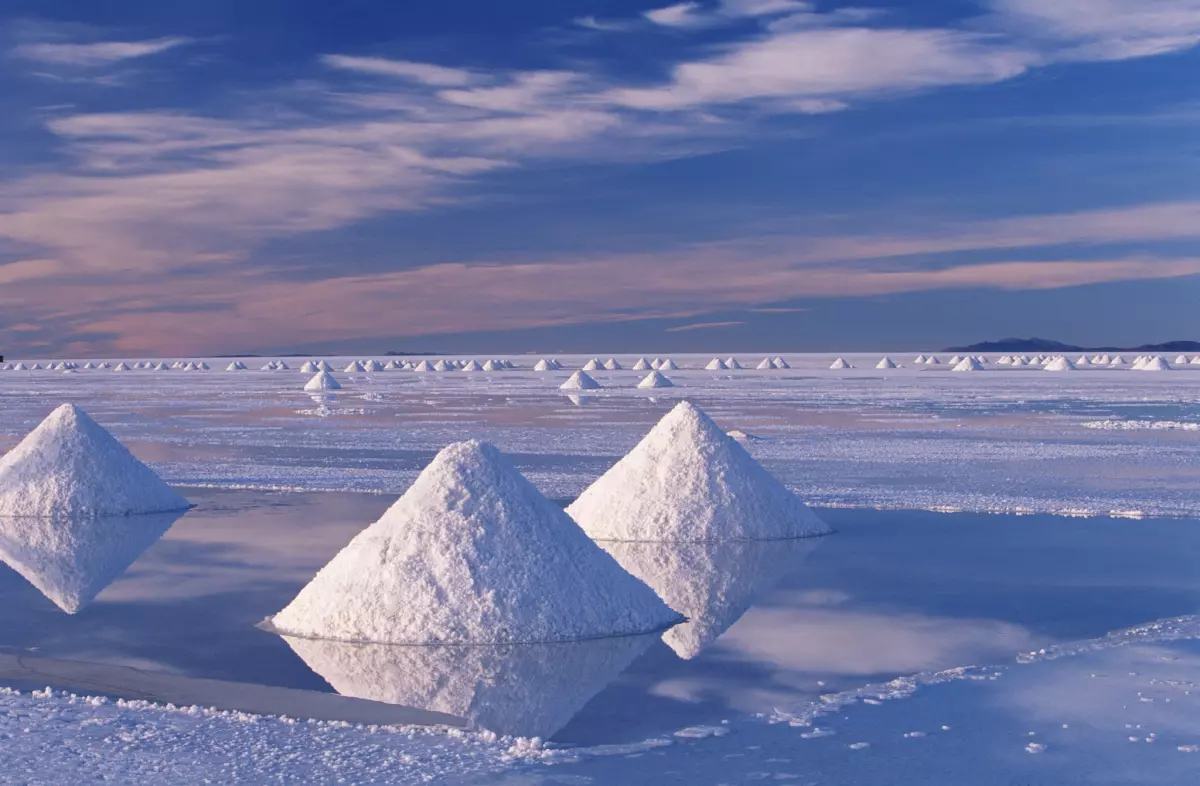
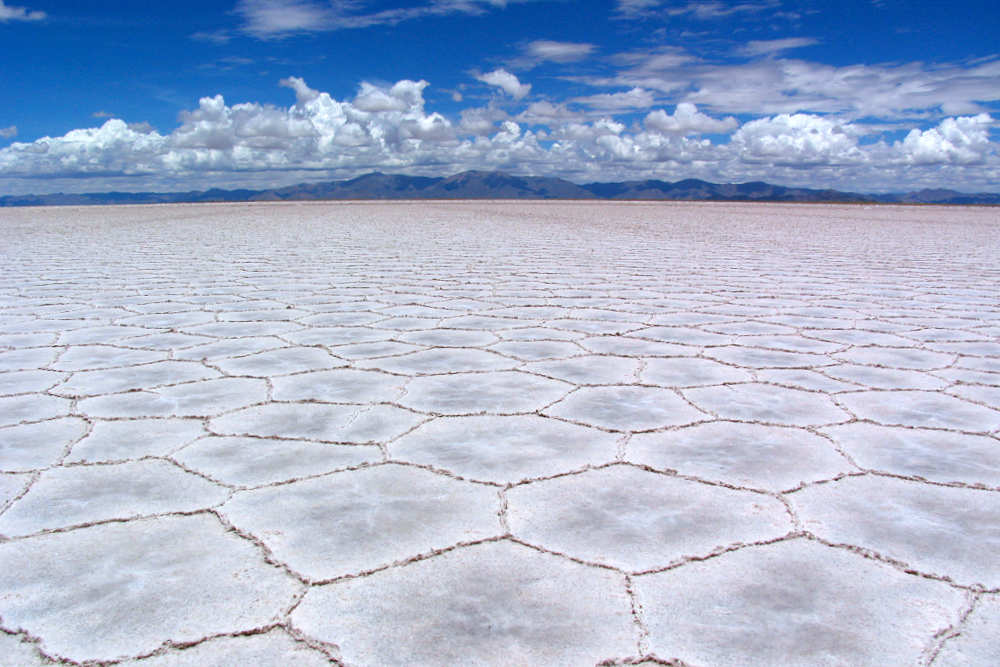
Cobalt and nickel are two of the other main materials required by lithium-ion batteries. These metals are rare, difficult to extract, and their production is fraught with health, safety, and environmental concerns. However, while these are problems now, technology is advancing quickly. Car manufacturers, including Tesla, are already producing EVs using batteries that incorporate alternatives to both metals.
While BEVs have undoubtedly played a crucial role in advancing clean transportation, their limitations are becoming increasingly apparent. As we delve deeper into the environmental and practical challenges posed by BEVs, it becomes clear that another solution is necessary. This is where hydrogen fuel cells can play an integral role in powering the next generation of EVs, offering a promising path forward.
The Hydrogen Advantage
To be clear, Hydrogen Fuel Cell Electric Vehicles (FCEVs) are not a silver bullet. They have their own limitations, like any other technology, but they offer some considerable advantages over standard battery EVs that cannot be ignored.
Hydrogen is a completely clean fuel
Like lithium-ion batteries, fuel cells produce electricity to power the vehicle. However, the hydrogen is not burned as fuel like gasoline. Instead, an electrochemical reaction between the hydrogen and the oxygen in the air generates electricity, which is constantly fed to an electric motor to power the car. The only byproducts of this process are water and heat. FCEVs still have a battery, but it is much smaller than those found in BEVs and receives energy from regenerative braking.
Unlike BEVs, which draw power from the grid and can strain grid infrastructure, FCEVs generate their own electricity through hydrogen. Since FCEVs do not rely on grid electricity, the type of power plant generating electricity for the grid becomes irrelevant. This means FCEVs do not add emissions by consuming electricity produced from fossil fuels, making them a more sustainable option without burdening the grid.
Refueling is quick and easy
Fuel cell electric cars can typically travel 500 kilometers or more on a full tank of hydrogen. Just like gasoline tanks, hydrogen tanks can be refueled in just 3 to 5 minutes. This rapid refueling process offers a level of convenience that battery electric vehicles currently cannot match, as BEVs require tens of minutes to several hours to recharge, depending on the charger and battery size.
Fuel cells are largely unaffected by extreme temperatures
Hydrogen fuel cells perform much better than batteries in extreme temperatures. They are designed to start and operate efficiently even in sub-zero temperatures. Modern fuel cell systems include features that handle cold starts effectively, making them suitable for harsh winter conditions. Although there might be a slight reduction in efficiency at extremely low temperatures, hydrogen fuel cells typically outperform lithium-ion batteries, which suffer significant capacity loss in the cold.
In hot climates, hydrogen fuel cells maintain their performance better than lithium-ion batteries, which can degrade quickly at high temperatures. Effective thermal management systems in fuel cell vehicles ensure that the cells operate within optimal temperature ranges, preventing overheating and maintaining efficiency.
Overall, hydrogen fuel cells provide a more consistent range across a wider temperature spectrum, making them a reliable option for varying climatic conditions. Their high energy density allows for longer ranges and faster refueling times, even in extreme temperatures, further solidifying their role as a crucial technology for the future of sustainable transportation.
Fuel cells last longer than batteries
While both hydrogen fuel cells and lithium-ion batteries degrade over time, the mechanisms and rates of degradation differ significantly. Hydrogen fuel cells typically have a longer operational life compared to lithium-ion batteries, often lasting for thousands of hours (or roughly 150,000-200,000 miles) before experiencing significant efficiency loss. Regular maintenance and the use of high-quality hydrogen can further extend their lifespan.
Conversely, lithium-ion batteries degrade primarily due to charge and discharge cycles and high temperatures, leading to a gradual loss of capacity and performance over time. EV batteries can easily lose about 6 percent of their capacity in the first 20,000 miles (32,000 km) and may lose around 20 percent of their original capacity after approximately 100,000 miles (160,000 km). This makes hydrogen fuel cells a more durable option for long-term sustainable transportation.
Fuel cells are lighter than batteries
In addition to their other advantages, fuel cells can also offer weight benefits. Hydrogen fuel cells generally provide a higher energy density than lithium-ion batteries, meaning they can store more energy per unit of weight. This results in a lighter overall system, which is particularly beneficial for applications requiring long ranges, such as heavy-duty vehicles and long-haul transportation.
The larger the vehicle, the greater the benefit of hydrogen becomes. While the weight difference may have a negligible impact on performance for passenger road vehicles, it becomes significant as the vehicle size increases. Batteries are too heavy to be used effectively on passenger planes, but hydrogen fuel cells are not, and they are already starting to be used in test flights.
In December 2022, Airbus, the world’s largest manufacturer of airliners, announced that it is developing a hydrogen-powered fuel cell engine for future passenger plane designs. These new hydrogen-powered passenger airliners could be in use by 2035, potentially revolutionizing air transportation, which accounts for 2.5% of worldwide carbon emissions—more than all of Germany combined.
Hydrogen is a high-potential technology with a specific energy-per-unit mass that is three times higher than traditional jet fuel.
- Airbus
City buses are already starting to use hydrogen fuel cells, and trains, freight trucks, cargo vessels, and more could also make the switch from fossil fuels to hydrogen. Cruise ships and cargo vessels could even be equipped with solar panels to produce their own hydrogen onboard, or more realistically, to reduce the load on the fuel cells for greater energy efficiency.
It’s estimated that a single shipping container vessel, the largest of which are almost half a kilometer long, can produce as much pollution as 50 million cars. With approximately 5,500 of these container ships at sea and about 55,000 merchant ships in total worldwide, hydrogen could play a pivotal role in curbing emissions from the shipping industry.
But are Hydrogen Fuel Cells safe?
While both hydrogen fuel cells and lithium-ion batteries are generally safe when properly engineered and maintained, hydrogen fuel cells offer distinct safety advantages. Unlike lithium-ion batteries, which are prone to thermal runaway and can catch fire or explode, hydrogen fuel cells are not susceptible to this phenomenon.
Today’s hydrogen fuel tanks are made from highly durable carbon fiber and designed to withstand both extreme pressure and extreme heat. For instance, Toyota weaves the carbon fiber into a triple-layered hydrogen tank that creates a bulletproof shell. They tested this by firing rifle rounds at a tank filled with pressurized hydrogen. The smaller caliber bullets simply bounced off. It took .50 calibre armour piercing rounds to penetrate the tank casing, and even then, it merely left a hole and the hydrogen gas leaked out.
When coupled with modern technology, hydrogen is safer than gasoline, and a leak lasts mere moments until the gas has harmlessly dispersed into the atmosphere. In contrast, when an EV battery catches fire, it can burn for hours and is extremely difficult to extinguish, requiring considerable firefighting resources.
Hydrogen storage systems are designed with robust safety features, including pressure relief devices and durable storage tanks. Additionally, if a hydrogen leak occurs, the gas dissipates quickly into the atmosphere, reducing the risk of fire or explosion. Moreover, the byproduct of hydrogen fuel cells is water, which is non-toxic, whereas damaged lithium-ion batteries can leak harmful chemicals. These safety benefits make hydrogen fuel cells an attractive option for sustainable transportation.
So, if hydrogen is better for the environment and doesn’t suffer the shortcomings faced by batteries, why aren’t we using hydrogen fuel cells everywhere already? What’s the catch?
The Downside of Hydrogen
While hydrogen fuel cells offer many advantages, they also come with significant challenges that need to be addressed.
Limited accessibility
Hydrogen is not readily available in its pure form and must be extracted from other substances, such as water and natural gas. Producing hydrogen using electrolysis—a process that uses electricity to separate the hydrogen from the oxygen in water—results in zero net carbon emissions but is inefficient. It takes about 50 kilowatt-hours (kWh) of energy to produce and compress just one kilogram of hydrogen from water.
To fill up the fuel cells in a Toyota Mirai, it takes about 5.6 kilograms of hydrogen, or approximately 280 kWh when using electrolysis. In comparison, it only takes about 50 kWh to fully charge a standard range Tesla Model 3. In 2023, a base Model 3 had an estimated range of about 437 kilometers (272 miles), while the Mirai had a range of about 647 kilometers (402 miles). This means it takes about 189 kWh—nearly four times the energy—for the Mirai to have an equivalent range to the Model 3.
Alternatively, extracting hydrogen from natural gas is more efficient, requiring only about 40 kWh of energy per kilogram of hydrogen, but this process can create carbon emissions, which need to be captured to maintain low emission levels. While efficiency is improving and costs are dropping, electrolysis remains more expensive than extracting hydrogen from fossil fuels.
Lack of infrastructure
One of the most significant hurdles for hydrogen fuel cell adoption is the lack of infrastructure. We currently do not have an extensive network of distribution pipelines and fueling stations for hydrogen. In contrast, battery chargers are becoming increasingly accessible, with significant investments from both government and private sectors. To promote hydrogen fuel cells, similar investments in infrastructure are necessary, encompassing everything from production facilities to distribution networks and refueling stations.
Higher costs and refueling limitations
Using hydrogen as a fuel is currently more expensive than electricity. The cost of producing, transporting, and storing hydrogen adds up, making it a less economical option compared to electric charging. Additionally, unlike BEVs, which can be conveniently recharged at home, hydrogen fuel cell vehicles require refueling at specialized stations, similar to gasoline-powered cars. This limitation adds another layer of inconvenience for potential users who may not have easy access to hydrogen refueling locations.
Conclusion
Despite these challenges, hydrogen fuel cells are critical for ending our dependence on fossil fuels and driving the adoption of electric vehicles, especially in the colder climates of North America, Europe, and East Asia. Their ability to perform well in extreme temperatures makes them a reliable option for regions that experience harsh winters and hot summers.
Moreover, hydrogen fuel cells offer significant environmental benefits, particularly as the world has not yet transitioned away from fossil fuel-based electricity generation. Since FCEVs generate their own electricity through hydrogen, they do not add emissions by consuming electricity produced from fossil fuels. This makes them a more sustainable option compared to BEVs, which currently rely on grid electricity that may still be derived from coal, natural gas, and other non-renewable sources.
Addressing the downsides of hydrogen fuel cells—through advancements in production efficiency, significant investments in infrastructure, and cost reduction strategies—will be crucial for realizing their full potential. By overcoming these obstacles, hydrogen fuel cells can complement battery electric vehicles, providing a diverse and robust solution to our global transportation needs, and playing a pivotal role in the fight against climate change.